Go 언어로 구현하는 컨테이너 오케스트레이션 시스템 🚀
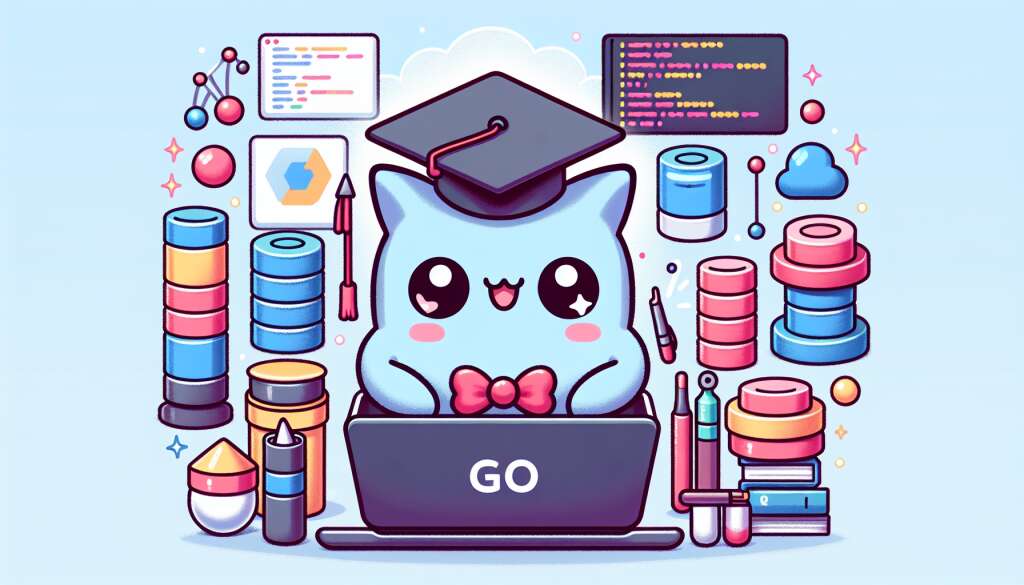
컨테이너 기술의 발전과 함께 오케스트레이션 시스템의 중요성이 날로 커지고 있습니다. 특히 Go 언어는 그 간결함과 강력한 동시성 모델로 인해 이러한 시스템을 구현하는 데 이상적인 선택이 되고 있죠. 이 글에서는 Go 언어를 사용하여 컨테이너 오케스트레이션 시스템을 구현하는 방법에 대해 상세히 알아보겠습니다. 🐳
컨테이너 오케스트레이션은 여러 컨테이너의 배포, 관리, 확장, 네트워킹을 자동화하는 프로세스를 말합니다. 이는 대규모 분산 시스템에서 특히 중요한데, 수백 또는 수천 개의 컨테이너를 효율적으로 관리해야 하기 때문이죠. Go 언어는 이러한 복잡한 시스템을 구현하는 데 적합한 여러 특성을 가지고 있습니다.
Go의 동시성 모델, 효율적인 메모리 관리, 빠른 컴파일 시간 등은 오케스트레이션 시스템 개발에 큰 장점이 됩니다. 또한, Go의 표준 라이브러리와 풍부한 생태계는 네트워킹, 로깅, 모니터링 등 오케스트레이션에 필요한 다양한 기능을 쉽게 구현할 수 있게 해줍니다.
이 글을 통해 여러분은 Go 언어를 사용하여 기본적인 컨테이너 오케스트레이션 시스템을 구현하는 방법을 배우게 될 것입니다. 컨테이너 생성부터 스케줄링, 로드 밸런싱, 서비스 디스커버리까지, 오케스트레이션의 핵심 개념들을 Go 코드로 구현해보며 이해할 수 있을 거예요. 💻
재능넷과 같은 플랫폼에서 활동하는 개발자들에게 이러한 지식은 매우 유용할 것입니다. 컨테이너 기술과 오케스트레이션에 대한 이해는 현대 소프트웨어 개발에서 중요한 역량이 되었기 때문이죠. 이 글을 통해 여러분의 기술적 역량을 한 단계 높일 수 있기를 바랍니다.
그럼 지금부터 Go 언어로 컨테이너 오케스트레이션 시스템을 구현하는 여정을 시작해볼까요? 🚀
1. Go 언어와 컨테이너 기술 소개 🐹
Go 언어는 Google에서 개발한 오픈소스 프로그래밍 언어로, 2009년에 처음 발표되었습니다. 간결한 문법, 강력한 동시성 지원, 빠른 컴파일 속도 등의 특징으로 인해 시스템 프로그래밍과 네트워크 프로그래밍 분야에서 큰 인기를 얻고 있습니다.
Go 언어의 주요 특징은 다음과 같습니다:
- 간결한 문법: Go는 최소한의 키워드와 간결한 문법 구조를 가지고 있어 학습과 사용이 쉽습니다.
- 정적 타입 시스템: 컴파일 시점에 타입 체크를 수행하여 런타임 에러를 줄일 수 있습니다.
- 고루틴(Goroutine)과 채널(Channel): Go의 동시성 모델은 가볍고 효율적인 고루틴과, 이들 간의 통신을 위한 채널을 제공합니다.
- 가비지 컬렉션: 자동 메모리 관리를 통해 개발자가 메모리 할당과 해제에 신경 쓰지 않아도 됩니다.
- 표준 라이브러리: 풍부한 표준 라이브러리를 제공하여 다양한 기능을 쉽게 구현할 수 있습니다.
이러한 특징들은 Go를 컨테이너 오케스트레이션 시스템 구현에 이상적인 언어로 만듭니다. 특히 동시성 처리와 네트워크 프로그래밍에 강점을 가지고 있어, 여러 컨테이너를 효율적으로 관리하고 통신하는 데 적합합니다.
한편, 컨테이너 기술은 애플리케이션과 그 의존성을 하나의 패키지로 묶어 이식성과 일관성을 제공하는 기술입니다. 컨테이너는 가상화 기술의 한 형태로, 운영체제 수준의 가상화를 제공합니다. 이는 전통적인 가상 머신에 비해 더 가볍고 빠르며, 리소스 효율성이 높습니다.
컨테이너 기술의 주요 이점은 다음과 같습니다:
- 이식성: 컨테이너는 어떤 환경에서도 동일하게 실행될 수 있습니다.
- 효율성: 컨테이너는 호스트 OS의 커널을 공유하여 리소스 사용을 최소화합니다.
- 격리: 각 컨테이너는 독립적인 환경에서 실행되어 보안과 안정성을 높입니다.
- 빠른 배포: 컨테이너는 빠르게 시작하고 중지할 수 있어 애플리케이션의 빠른 배포와 확장이 가능합니다.
이러한 컨테이너 기술의 장점을 최대한 활용하기 위해서는 효율적인 오케스트레이션 시스템이 필요합니다. 여기서 Go 언어의 강점이 빛을 발하게 되는 것이죠.
Go 언어와 컨테이너 기술의 조합은 현대적인 클라우드 네이티브 애플리케이션 개발에 있어 매우 강력한 도구가 됩니다. Go의 효율성과 컨테이너의 이식성이 만나 확장 가능하고 관리하기 쉬운 시스템을 구축할 수 있게 해주는 것입니다.
다음 섹션에서는 이러한 Go 언어와 컨테이너 기술을 바탕으로, 실제로 간단한 컨테이너 오케스트레이션 시스템을 구현하는 방법에 대해 알아보겠습니다. 🛠️
2. 컨테이너 오케스트레이션 시스템의 기본 구조 설계 🏗️
컨테이너 오케스트레이션 시스템을 구현하기 위해서는 먼저 전체적인 구조를 설계해야 합니다. 이 시스템은 여러 개의 핵심 컴포넌트로 구성되며, 각 컴포넌트는 특정 역할을 담당합니다. 우리가 구현할 기본적인 오케스트레이션 시스템의 주요 컴포넌트는 다음과 같습니다:
- Scheduler (스케줄러): 컨테이너를 어떤 노드에 배치할지 결정합니다.
- Node Manager (노드 관리자): 각 노드의 상태를 관리하고 모니터링합니다.
- Container Manager (컨테이너 관리자): 컨테이너의 생명주기를 관리합니다.
- Network Manager (네트워크 관리자): 컨테이너 간 네트워크를 설정하고 관리합니다.
- API Server (API 서버): 사용자와 다른 시스템 컴포넌트가 상호작용할 수 있는 인터페이스를 제공합니다.
이제 각 컴포넌트에 대해 자세히 살펴보고, Go 언어로 어떻게 구현할 수 있는지 알아보겠습니다.
2.1 Scheduler (스케줄러) 🗓️
스케줄러는 오케스트레이션 시스템의 핵심 컴포넌트입니다. 새로운 컨테이너를 배치할 때 어떤 노드가 가장 적합한지를 결정하는 역할을 합니다. 스케줄링 결정은 여러 요소를 고려하여 이루어집니다:
- 노드의 가용 리소스 (CPU, 메모리 등)
- 네트워크 토폴로지
- 컨테이너의 요구사항
- 현재 노드의 워크로드
Go로 스케줄러를 구현할 때는 다음과 같은 구조를 사용할 수 있습니다:
type Scheduler struct {
nodes []*Node
strategy SchedulingStrategy
}
type SchedulingStrategy interface {
SelectNode(container *Container, nodes []*Node) *Node
}
func (s *Scheduler) Schedule(container *Container) *Node {
return s.strategy.SelectNode(container, s.nodes)
}
이 구조에서 SchedulingStrategy
인터페이스를 사용하여 다양한 스케줄링 알고리즘을 쉽게 구현하고 교체할 수 있습니다. 예를 들어, 간단한 라운드 로빈 전략은 다음과 같이 구현할 수 있습니다:
type RoundRobinStrategy struct {
lastIndex int
}
func (r *RoundRobinStrategy) SelectNode(container *Container, nodes []*Node) *Node {
r.lastIndex = (r.lastIndex + 1) % len(nodes)
return nodes[r.lastIndex]
}
2.2 Node Manager (노드 관리자) 🖥️
노드 관리자는 클러스터 내의 모든 노드의 상태를 추적하고 관리합니다. 각 노드의 리소스 사용량, 건강 상태, 실행 중인 컨테이너 등의 정보를 유지합니다. Go로 노드 관리자를 구현하는 기본 구조는 다음과 같습니다:
type NodeManager struct {
nodes map[string]*Node
mu sync.RWMutex
}
type Node struct {
ID string
Capacity Resources
Used Resources
Containers map[string]*Container
}
type Resources struct {
CPU int
Memory int
}
func (nm *NodeManager) AddNode(node *Node) {
nm.mu.Lock()
defer nm.mu.Unlock()
nm.nodes[node.ID] = node
}
func (nm *NodeManager) RemoveNode(nodeID string) {
nm.mu.Lock()
defer nm.mu.Unlock()
delete(nm.nodes, nodeID)
}
func (nm *NodeManager) GetNode(nodeID string) (*Node, bool) {
nm.mu.RLock()
defer nm.mu.RUnlock()
node, exists := nm.nodes[nodeID]
return node, exists
}
이 구조에서는 sync.RWMutex
를 사용하여 동시성 문제를 해결하고 있습니다. 여러 고루틴이 동시에 노드 정보에 접근할 때 발생할 수 있는 경쟁 조건을 방지합니다.
2.3 Container Manager (컨테이너 관리자) 📦
컨테이너 관리자는 개별 컨테이너의 생명주기를 관리합니다. 컨테이너의 생성, 시작, 중지, 삭제 등의 작업을 처리합니다. 또한 컨테이너의 상태를 모니터링하고, 필요한 경우 재시작 등의 작업을 수행합니다.
type ContainerManager struct {
containers map[string]*Container
mu sync.RWMutex
}
type Container struct {
ID string
Image string
Status ContainerStatus
Node *Node
}
type ContainerStatus int
const (
Created ContainerStatus = iota
Running
Stopped
Failed
)
func (cm *ContainerManager) CreateContainer(image string) (*Container, error) {
// 컨테이너 생성 로직
}
func (cm *ContainerManager) StartContainer(containerID string) error {
// 컨테이너 시작 로직
}
func (cm *ContainerManager) StopContainer(containerID string) error {
// 컨테이너 중지 로직
}
func (cm *ContainerManager) DeleteContainer(containerID string) error {
// 컨테이너 삭제 로직
}
실제 구현에서는 컨테이너 런타임 (예: Docker)과 통신하는 로직이 각 메서드에 포함되어야 합니다. Go의 os/exec
패키지를 사용하여 Docker CLI 명령을 실행하거나, Docker API 클라이언트 라이브러리를 사용할 수 있습니다.
2.4 Network Manager (네트워크 관리자) 🌐
네트워크 관리자는 컨테이너 간의 네트워크 연결을 설정하고 관리합니다. 이는 컨테이너 간 통신, 로드 밸런싱, 서비스 디스커버리 등의 기능을 포함합니다.
type NetworkManager struct {
networks map[string]*Network
mu sync.RWMutex
}
type Network struct {
ID string
Name string
Subnet string
Gateway string
}
func (nm *NetworkManager) CreateNetwork(name, subnet, gateway string) (*Network, error) {
// 네트워크 생성 로직
}
func (nm *NetworkManager) DeleteNetwork(networkID string) error {
// 네트워크 삭제 로직
}
func (nm *NetworkManager) ConnectContainerToNetwork(containerID, networkID string) error {
// 컨테이너를 네트워크에 연결하는 로직
}
func (nm *NetworkManager) DisconnectContainerFromNetwork(containerID, networkID string) error {
// 컨테이너를 네트워크에서 분리하는 로직
}
네트워크 관리자의 실제 구현에는 리눅스 네트워크 네임스페이스, 브릿지, iptables 등을 조작하는 복잡한 로직이 포함될 수 있습니다. Go의 netlink
패키지를 사용하여 이러한 저수준 네트워킹 작업을 수행할 수 있습니다.
2.5 API Server (API 서버) 🚀
API 서버는 사용자와 다른 시스템 컴포넌트가 오케스트레이션 시스템과 상호작용할 수 있는 인터페이스를 제공합니다. RESTful API를 구현하여 컨테이너와 노드의 관리, 모니터링 등의 기능을 제공할 수 있습니다.
type APIServer struct {
scheduler *Scheduler
nodeManager *NodeManager
containerManager *ContainerManager
networkManager *NetworkManager
}
func (s *APIServer) Start() error {
http.HandleFunc("/containers", s.handleContainers)
http.HandleFunc("/nodes", s.handleNodes)
http.HandleFunc("/networks", s.handleNetworks)
return http.ListenAndServe(":8080", nil)
}
func (s *APIServer) handleContainers(w http.ResponseWriter, r *http.Request) {
// 컨테이너 관련 API 핸들러
}
func (s *APIServer) handleNodes(w http.ResponseWriter, r *http.Request) {
// 노드 관련 API 핸들러
}
func (s *APIServer) handleNetworks(w http.ResponseWriter, r *http.Request) {
// 네트워크 관련 API 핸들러
}
이러한 기본 구조를 바탕으로, 각 컴포넌트의 세부 기능을 구현하고 이들을 연결하여 완전한 오케스트레이션 시스템을 만들 수 있습니다. 다음 섹션에서는 이러한 컴포넌트들의 구체적인 구현 방법에 대해 더 자세히 알아보겠습니다. 🛠️
이러한 구조를 바탕으로 Go 언어의 강력한 동시성 모델과 효율적인 메모리 관리를 활용하여, 확장 가능하고 성능이 뛰어난 컨테이너 오케스트레이션 시스템을 구축할 수 있습니다. 다음 섹션에서는 각 컴포넌트의 세부적인 구현 방법과 이들을 어떻게 통합하는지에 대해 더 자세히 살펴보겠습니다. 💪
3. 스케줄러 구현하기 🗓️
스케줄러는 오케스트레이션 시스템의 핵심 컴포넌트로, 새로운 컨테이너를 어떤 노드에 배치할지 결정하는 중요한 역할을 합니다. 효율적인 스케줄러를 구현하기 위해서는 다양한 요소를 고려해야 합니다. 여기서는 간단한 스케줄러를 구현하고, 점진적으로 기능을 확장해 나가는 방법을 살펴보겠습니다.
3.1 기본 스케줄러 구조
먼저, 기본적인 스케줄러 구조를 정의해보겠습니다:
type Scheduler struct {
nodes []*Node
strategy SchedulingStrategy
}
type SchedulingStrategy interface {
SelectNode(container *Container, nodes []*Node) *Node
}
func NewScheduler(strategy SchedulingStrategy) *Scheduler {
return &Scheduler{
nodes: make([]*Node, 0),
strategy: strategy,
}
}
func (s *Scheduler) AddNode(node *Node) {
s.nodes = append(s.nodes, node)
}
func (s *Scheduler) Schedule(container *Container) *Node {
return s.strategy.SelectNode(container, s.nodes)
}
이 구조에서 SchedulingStrategy
인터페이스를 사용하여 다양한 스케줄링 알고리즘을 쉽게 구현하고 교체할 수 있도록 했습니다.
3.2 라운드 로빈 스케줄링 전략
가장 간단한 스케줄링 전략 중 하나인 라운드 로빈 방식을 구현해보겠습니다:
type RoundRobinStrategy struct {
lastIndex int
}
func (r *RoundRobinStrategy) SelectNode(container *Container, nodes []*Node) *Node {
if len(nodes) == 0 {
return nil
}
r.lastIndex = (r.lastIndex + 1) % len(nodes)
return nodes[r.lastIndex]
}
이 전략은 단순히 노드 리스트를 순환하면서 컨테이너를 배치합니다. 간단하지만 노드의 현재 상태를 고려하지 않는다는 단점이 있습니다.
3.3 리소스 기반 스케줄링 전략
좀 더 발전된 형태로, 각 노드의 가용 리소스를 고려하는 스케줄링 전략을 구현해보겠습니다:
type ResourceBasedStrategy struct{}
func (r *ResourceBasedStrategy) SelectNode(container *Container, nodes []*Node) *Node {
var selectedNode *Node
maxAvailableResource := 0
for _, node := range nodes {
availableResource := node.Capacity.CPU - node.Used.CPU
if availableResource > maxAvailableResource {
maxAvailableResource = availableResource
selectedNode = node
}
}
return selectedNode
}
이 전략은 가장 많은 가용 CPU를 가진 노드를 선택합니다. 실제 구현에서는 CPU뿐만 아니라 메모리, 디스크 공간 등 다양한 리소스를 고려해야 합니다.
3.4 affinity 및 anti-affinity 규칙 구현
더 복잡한 스케줄링 요구사항을 처리하기 위해 affinity 및 anti-affinity 규칙을 구현할 수 있습니다:
type AffinityRule struct {
LabelKey string
LabelValue string
}
type AntiAffinityRule struct { AffinityRule과 동일한 구조를 가집니다.
}
type AffinityBasedStrategy struct {
affinityRules []AffinityRule
antiAffinityRules []AntiAffinityRule
}
func (a *AffinityBasedStrategy) SelectNode(container *Container, nodes []*Node) *Node {
var candidateNodes []*Node
// Affinity 규칙 적용
for _, node := range nodes {
if a.matchesAffinityRules(node) {
candidateNodes = append(candidateNodes, node)
}
}
// Anti-affinity 규칙 적용
candidateNodes = a.filterByAntiAffinityRules(candidateNodes)
// 남은 후보 노드 중에서 리소스 기반으로 선택
return a.selectByResource(container, candidateNodes)
}
func (a *AffinityBasedStrategy) matchesAffinityRules(node *Node) bool {
for _, rule := range a.affinityRules {
if value, exists := node.Labels[rule.LabelKey]; !exists || value != rule.LabelValue {
return false
}
}
return true
}
func (a *AffinityBasedStrategy) filterByAntiAffinityRules(nodes []*Node) []*Node {
var filteredNodes []*Node
for _, node := range nodes {
if !a.violatesAntiAffinityRules(node) {
filteredNodes = append(filteredNodes, node)
}
}
return filteredNodes
}
func (a *AffinityBasedStrategy) violatesAntiAffinityRules(node *Node) bool {
for _, rule := range a.antiAffinityRules {
if value, exists := node.Labels[rule.LabelKey]; exists && value == rule.LabelValue {
return true
}
}
return false
}
func (a *AffinityBasedStrategy) selectByResource(container *Container, nodes []*Node) *Node {
// 리소스 기반 선택 로직 (이전의 ResourceBasedStrategy와 유사)
// ...
}
이 전략은 먼저 affinity 규칙에 맞는 노드들을 선별한 후, anti-affinity 규칙을 위반하는 노드들을 제외합니다. 그리고 남은 후보 노드들 중에서 리소스 상태를 고려하여 최종 노드를 선택합니다.
3.5 스케줄러 성능 최적화
대규모 클러스터에서 스케줄러의 성능은 매우 중요합니다. 다음과 같은 방법으로 스케줄러의 성능을 최적화할 수 있습니다:
- 병렬 처리: Go의 고루틴을 활용하여 여러 컨테이너를 동시에 스케줄링합니다.
- 캐싱: 노드의 상태 정보를 캐싱하여 반복적인 계산을 줄입니다.
- 인덱싱: 노드와 컨테이너의 특성에 따른 인덱스를 만들어 빠른 검색이 가능하게 합니다.
병렬 처리를 적용한 스케줄러의 예시 코드는 다음과 같습니다:
func (s *Scheduler) ScheduleMultiple(containers []*Container) map[*Container]*Node {
result := make(map[*Container]*Node)
var wg sync.WaitGroup
var mu sync.Mutex
for _, container := range containers {
wg.Add(1)
go func(c *Container) {
defer wg.Done()
node := s.Schedule(c)
mu.Lock()
result[c] = node
mu.Unlock()
}(container)
}
wg.Wait()
return result
}
이 구현에서는 각 컨테이너의 스케줄링을 별도의 고루틴에서 처리하여 병렬성을 높였습니다. sync.WaitGroup
을 사용하여 모든 스케줄링이 완료될 때까지 기다리고, sync.Mutex
를 사용하여 결과 맵에 대한 동시 접근을 제어합니다.
3.6 스케줄러 테스트
스케줄러의 정확성과 성능을 검증하기 위해 단위 테스트와 벤치마크 테스트를 작성하는 것이 중요합니다. 다음은 간단한 테스트 코드 예시입니다:
func TestRoundRobinStrategy(t *testing.T) {
nodes := []*Node{
{ID: "node1"},
{ID: "node2"},
{ID: "node3"},
}
strategy := &RoundRobinStrategy{}
scheduler := NewScheduler(strategy)
scheduler.nodes = nodes
container := &Container{}
selectedNode1 := scheduler.Schedule(container)
selectedNode2 := scheduler.Schedule(container)
selectedNode3 := scheduler.Schedule(container)
selectedNode4 := scheduler.Schedule(container)
if selectedNode1.ID != "node1" || selectedNode2.ID != "node2" ||
selectedNode3.ID != "node3" || selectedNode4.ID != "node1" {
t.Errorf("RoundRobinStrategy did not cycle through nodes correctly")
}
}
func BenchmarkScheduler(b *testing.B) {
nodes := make([]*Node, 1000)
for i := range nodes {
nodes[i] = &Node{ID: fmt.Sprintf("node%d", i)}
}
strategy := &ResourceBasedStrategy{}
scheduler := NewScheduler(strategy)
scheduler.nodes = nodes
container := &Container{}
b.ResetTimer()
for i := 0; i < b.N; i++ {
scheduler.Schedule(container)
}
}
이러한 테스트를 통해 스케줄러의 동작을 검증하고 성능을 측정할 수 있습니다.
스케줄러는 오케스트레이션 시스템의 효율성과 확장성을 결정짓는 핵심 컴포넌트입니다. 여기서 소개한 기본적인 구현을 바탕으로, 실제 환경에서의 요구사항과 제약 조건을 고려하여 더욱 정교한 스케줄링 알고리즘을 개발할 수 있습니다. 다음 섹션에서는 노드 관리자의 구현에 대해 살펴보겠습니다. 🚀
4. 노드 관리자 구현하기 🖥️
노드 관리자는 클러스터 내의 모든 노드의 상태를 추적하고 관리하는 중요한 컴포넌트입니다. 이 섹션에서는 Go 언어를 사용하여 효율적이고 확장 가능한 노드 관리자를 구현하는 방법을 살펴보겠습니다.
4.1 기본 구조 정의
먼저 노드와 노드 관리자의 기본 구조를 정의해보겠습니다:
type Node struct {
ID string
IP string
Capacity Resources
Used Resources
Available Resources
Status NodeStatus
Labels map[string]string
Containers map[string]*Container
}
type Resources struct {
CPU int64
Memory int64
Disk int64
}
type NodeStatus int
const (
NodeStatusReady NodeStatus = iota
NodeStatusNotReady
NodeStatusMaintenance
)
type NodeManager struct {
nodes map[string]*Node
mu sync.RWMutex
}
func NewNodeManager() *NodeManager {
return &NodeManager{
nodes: make(map[string]*Node),
}
}
4.2 노드 관리 기능 구현
노드 관리자의 주요 기능을 구현해보겠습니다:
func (nm *NodeManager) AddNode(node *Node) error {
nm.mu.Lock()
defer nm.mu.Unlock()
if _, exists := nm.nodes[node.ID]; exists {
return fmt.Errorf("node with ID %s already exists", node.ID)
}
nm.nodes[node.ID] = node
return nil
}
func (nm *NodeManager) RemoveNode(nodeID string) error {
nm.mu.Lock()
defer nm.mu.Unlock()
if _, exists := nm.nodes[nodeID]; !exists {
return fmt.Errorf("node with ID %s does not exist", nodeID)
}
delete(nm.nodes, nodeID)
return nil
}
func (nm *NodeManager) GetNode(nodeID string) (*Node, error) {
nm.mu.RLock()
defer nm.mu.RUnlock()
node, exists := nm.nodes[nodeID]
if !exists {
return nil, fmt.Errorf("node with ID %s does not exist", nodeID)
}
return node, nil
}
func (nm *NodeManager) UpdateNodeStatus(nodeID string, status NodeStatus) error {
nm.mu.Lock()
defer nm.mu.Unlock()
node, exists := nm.nodes[nodeID]
if !exists {
return fmt.Errorf("node with ID %s does not exist", nodeID)
}
node.Status = status
return nil
}
func (nm *NodeManager) UpdateNodeResources(nodeID string, used Resources) error {
nm.mu.Lock()
defer nm.mu.Unlock()
node, exists := nm.nodes[nodeID]
if !exists {
return fmt.Errorf("node with ID %s does not exist", nodeID)
}
node.Used = used
node.Available = Resources{
CPU: node.Capacity.CPU - used.CPU,
Memory: node.Capacity.Memory - used.Memory,
Disk: node.Capacity.Disk - used.Disk,
}
return nil
}
4.3 노드 모니터링 구현
노드의 상태를 주기적으로 모니터링하는 기능을 구현해보겠습니다:
func (nm *NodeManager) StartMonitoring(interval time.Duration) {
ticker := time.NewTicker(interval)
go func() {
for range ticker.C {
nm.monitorNodes()
}
}()
}
func (nm *NodeManager) monitorNodes() {
nm.mu.RLock()
nodeIDs := make([]string, 0, len(nm.nodes))
for id := range nm.nodes {
nodeIDs = append(nodeIDs, id)
}
nm.mu.RUnlock()
var wg sync.WaitGroup
for _, id := range nodeIDs {
wg.Add(1)
go func(nodeID string) {
defer wg.Done()
nm.checkNodeHealth(nodeID)
}(id)
}
wg.Wait()
}
func (nm *NodeManager) checkNodeHealth(nodeID string) {
// 실제 구현에서는 노드에 health check 요청을 보내고 응답을 확인합니다.
// 여기서는 간단한 예시로 대체합니다.
isHealthy := rand.Float32() < 0.95 // 95% 확률로 healthy
var newStatus NodeStatus
if isHealthy {
newStatus = NodeStatusReady
} else {
newStatus = NodeStatusNotReady
}
nm.UpdateNodeStatus(nodeID, newStatus)
}
4.4 노드 선택 알고리즘 구현
스케줄러와 연동하여 사용할 수 있는 노드 선택 알고리즘을 구현해보겠습니다:
func (nm *NodeManager) GetAvailableNodes() []*Node {
nm.mu.RLock()
defer nm.mu.RUnlock()
availableNodes := make([]*Node, 0)
for _, node := range nm.nodes {
if node.Status == NodeStatusReady && node.Available.CPU > 0 && node.Available.Memory > 0 {
availableNodes = append(availableNodes, node)
}
}
return availableNodes
}
func (nm *NodeManager) SelectNodeForContainer(container *Container) (*Node, error) {
availableNodes := nm.GetAvailableNodes()
if len(availableNodes) == 0 {
return nil, fmt.Errorf("no available nodes")
}
// 여기서는 간단히 첫 번째 가용 노드를 선택합니다.
// 실제 구현에서는 더 복잡한 선택 알고리즘을 사용할 수 있습니다.
return availableNodes[0], nil
}
4.5 노드 레이블 관리
노드에 레이블을 추가하고 관리하는 기능을 구현해보겠습니다:
func (nm *NodeManager) AddNodeLabel(nodeID, key, value string) error {
nm.mu.Lock()
defer nm.mu.Unlock()
node, exists := nm.nodes[nodeID]
if !exists {
return fmt.Errorf("node with ID %s does not exist", nodeID)
}
if node.Labels == nil {
node.Labels = make(map[string]string)
}
node.Labels[key] = value
return nil
}
func (nm *NodeManager) RemoveNodeLabel(nodeID, key string) error {
nm.mu.Lock()
defer nm.mu.Unlock()
node, exists := nm.nodes[nodeID]
if !exists {
return fmt.Errorf("node with ID %s does not exist", nodeID)
}
if node.Labels != nil {
delete(node.Labels, key)
}
return nil
}
func (nm *NodeManager) GetNodesByLabel(key, value string) []*Node {
nm.mu.RLock()
defer nm.mu.RUnlock()
var matchingNodes []*Node
for _, node := range nm.nodes {
if labelValue, exists := node.Labels[key]; exists && labelValue == value {
matchingNodes = append(matchingNodes, node)
}
}
return matchingNodes
}
4.6 노드 관리자 테스트
노드 관리자의 기능을 검증하기 위한 테스트 코드를 작성해보겠습니다:
func TestNodeManager(t *testing.T) {
nm := NewNodeManager()
// 노드 추가 테스트
node1 := &Node{ID: "node1", IP: "192.168.1.1", Capacity: Resources{CPU: 4, Memory: 8192, Disk: 100000}}
err := nm.AddNode(node1)
if err != nil {
t.Errorf("Failed to add node: %v", err)
}
// 노드 조회 테스트
retrievedNode, err := nm.GetNode("node1")
if err != nil {
t.Errorf("Failed to get node: %v", err)
}
if retrievedNode.ID != "node1" {
t.Errorf("Retrieved node ID does not match: expected node1, got %s", retrievedNode.ID)
}
// 노드 상태 업데이트 테스트
err = nm.UpdateNodeStatus("node1", NodeStatusMaintenance)
if err != nil {
t.Errorf("Failed to update node status: %v", err)
}
updatedNode, _ := nm.GetNode("node1")
if updatedNode.Status != NodeStatusMaintenance {
t.Errorf("Node status not updated correctly: expected Maintenance, got %v", updatedNode.Status)
}
// 노드 레이블 추가 테스트
err = nm.AddNodeLabel("node1", "env", "production")
if err != nil {
t.Errorf("Failed to add node label: %v", err)
}
labeledNode, _ := nm.GetNode("node1")
if labeledNode.Labels["env"] != "production" {
t.Errorf("Node label not added correctly: expected production, got %s", labeledNode.Labels["env"])
}
// 노드 제거 테스트
err = nm.RemoveNode("node1")
if err != nil {
t.Errorf("Failed to remove node: %v", err)
}
_, err = nm.GetNode("node1")
if err == nil {
t.Errorf("Node not removed correctly")
}
}
이러한 테스트를 통해 노드 관리자의 주요 기능들이 정상적으로 동작하는지 확인할 수 있습니다.
노드 관리자는 오케스트레이션 시스템의 중요한 구성 요소로, 클러스터의 전반적인 상태를 관리하고 모니터링하는 역할을 합니다. 여기서 구현한 기본적인 기능들을 바탕으로, 실제 환경에서의 요구사항에 맞춰 더욱 복잡하고 강력한 노드 관리 시스템을 개발할 수 있습니다. 다음 섹션에서는 컨테이너 관리자의 구현에 대해 살펴보겠습니다. 🚀
5. 컨테이너 관리자 구현하기 📦
컨테이너 관리자는 개별 컨테이너의 생명주기를 관리하는 핵심 컴포넌트입니다. 이 섹션에서는 Go 언어를 사용하여 효율적이고 확장 가능한 컨테이너 관리자를 구현하는 방법을 살펴보겠습니다.
5.1 기본 구조 정의
먼저 컨테이너와 컨테이너 관리자의 기본 구조를 정의해보겠습니다:
type Container struct {
ID string
Name string
Image string
Status ContainerStatus
Node *Node
Resources Resources
Ports map[int]int
Env map[string]string
}
type ContainerStatus int
const (
ContainerStatusCreated ContainerStatus = iota
ContainerStatusRunning
ContainerStatusPaused
ContainerStatusStopped
ContainerStatusExited
)
type ContainerManager struct {
containers map[string]*Container
mu sync.RWMutex
}
func NewContainerManager() *ContainerManager {
return &ContainerManager{
containers: make(map[string]*Container),
}
}
5.2 컨테이너 관리 기능 구현
컨테이너 관리자의 주요 기능을 구현해보겠습니다:
func (cm *ContainerManager) CreateContainer(spec ContainerSpec) (*Container, error) {
cm.mu.Lock()
defer cm.mu.Unlock()
// 컨테이너 ID 생성
id := generateUniqueID()
container := &Container{
ID: id,
Name: spec.Name,
Image: spec.Image,
Status: ContainerStatusCreated,
Resources: spec.Resources,
Ports: spec.Ports,
Env: spec.Env,
}
cm.containers[id] = container
return container, nil
}
func (cm *ContainerManager) StartContainer(id string) error {
cm.mu.Lock()
defer cm.mu.Unlock()
container, exists := cm.containers[id]
if !exists {
return fmt.Errorf("container with ID %s does not exist", id)
}
// 여기에 실제 컨테이너 시작 로직 구현
// 예: Docker API 호출
container.Status = ContainerStatusRunning
return nil
}
func (cm *ContainerManager) StopContainer(id string) error {
cm.mu.Lock()
defer cm.mu.Unlock()
container, exists := cm.containers[id]
if !exists {
return fmt.Errorf("container with ID %s does not exist", id)
}
// 여기에 실제 컨테이너 중지 로직 구현
// 예: Docker API 호출
container.Status = ContainerStatusStopped
return nil
}
func (cm *ContainerManager) RemoveContainer(id string) error {
cm.mu.Lock()
defer cm.mu.Unlock()
if _, exists := cm.containers[id]; !exists {
return fmt.Errorf("container with ID %s does not exist", id)
}
// 여기에 실제 컨테이너 제거 로직 구현
// 예: Docker API 호출
delete(cm.containers, id)
return nil
}
func (cm *ContainerManager) GetContainer(id string) (*Container, error) {
cm.mu.RLock()
defer cm.mu.RUnlock()
container, exists := cm.containers[id]
if !exists {
return nil, fmt.Errorf("container with ID %s does not exist", id)
}
return container, nil
}
func (cm *ContainerManager) ListContainers() []*Container {
cm.mu.RLock()
defer cm.mu.RUnlock()
containers := make([]*Container, 0, len(cm.containers))
for _, container := range cm.containers {
containers = append(containers, container)
}
return containers
}