C++로 시스템 프로그래밍 시작하기: 운영체제 핵심 개념 구현 🖥️💻
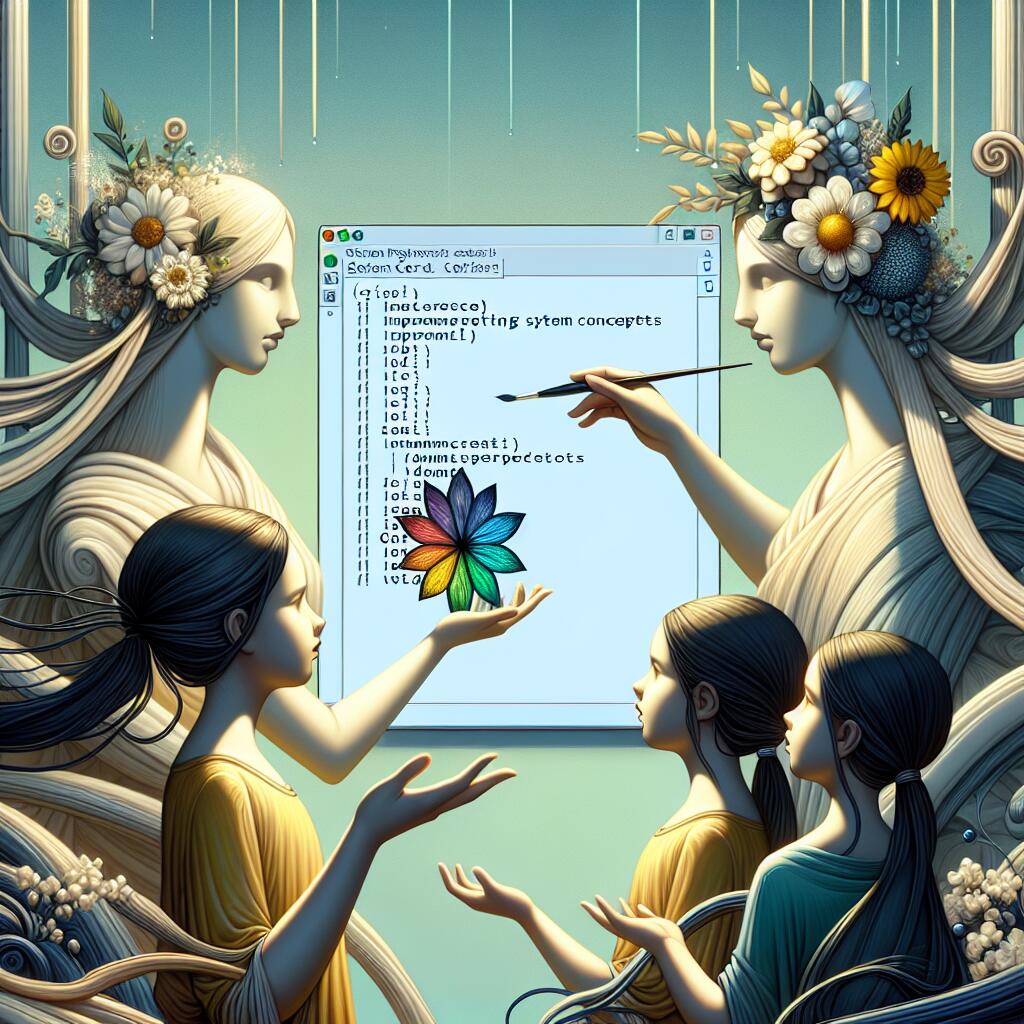
시스템 프로그래밍은 컴퓨터 과학의 핵심 분야 중 하나로, 운영체제와 하드웨어 간의 상호작용을 다루는 중요한 영역입니다. C++는 이러한 시스템 프로그래밍에 적합한 언어로 널리 사용되고 있죠. 오늘은 C++를 활용하여 운영체제의 핵심 개념들을 직접 구현해보면서, 시스템 프로그래밍의 세계로 깊이 들어가 보겠습니다. 🚀
이 글을 통해 여러분은 단순히 이론적인 지식을 넘어, 실제로 동작하는 코드를 작성하며 운영체제의 핵심 메커니즘을 이해할 수 있을 것입니다. 마치 재능넷에서 다양한 재능을 거래하듯, 우리는 여기서 C++와 시스템 프로그래밍의 재능을 습득하고 공유하게 될 것입니다. 자, 그럼 시작해볼까요? 🎨✨
1. 프로세스 관리: 멀티태스킹의 기초 🔄
운영체제의 가장 기본적인 기능 중 하나는 프로세스 관리입니다. 여러 프로그램을 동시에 실행하는 것처럼 보이게 하는 멀티태스킹의 핵심이죠. C++로 간단한 프로세스 관리 시스템을 구현해보겠습니다.
#include <iostream>
#include <vector>
#include <queue>
#include <string>
class Process {
public:
Process(int id, std::string name, int priority) : id(id), name(name), priority(priority) {}
int getId() const { return id; }
std::string getName() const { return name; }
int getPriority() const { return priority; }
private:
int id;
std::string name;
int priority;
};
class ProcessManager {
public:
void addProcess(const Process& process) {
processes.push(process);
}
void runNextProcess() {
if (!processes.empty()) {
Process current = processes.top();
processes.pop();
std::cout << "Running process: " << current.getName() << " (ID: " << current.getId() << ")" << std::endl;
} else {
std::cout << "No processes to run." << std::endl;
}
}
private:
std::priority_queue<process std::vector>, std::function<bool process>> processes{
[](const Process& a, const Process& b) { return a.getPriority() < b.getPriority(); }
};
};
int main() {
ProcessManager manager;
manager.addProcess(Process(1, "Web Browser", 3));
manager.addProcess(Process(2, "Text Editor", 2));
manager.addProcess(Process(3, "File Manager", 1));
for (int i = 0; i < 3; ++i) {
manager.runNextProcess();
}
return 0;
}
</bool></process>
이 코드에서는 우선순위 큐를 사용하여 간단한 프로세스 스케줄러를 구현했습니다. 각 프로세스는 ID, 이름, 우선순위를 가지며, 우선순위가 높은 프로세스가 먼저 실행됩니다. 이는 실제 운영체제의 프로세스 스케줄링 알고리즘을 간소화한 버전이라고 볼 수 있죠.
2. 메모리 관리: 동적 메모리 할당 구현 💾
메모리 관리는 운영체제의 핵심 기능 중 하나입니다. 여기서는 간단한 메모리 할당기를 구현해보겠습니다. 이 할당기는 First-Fit 알고리즘을 사용하여 메모리 블록을 할당합니다.
#include <iostream>
#include <vector>
#include <algorithm>
struct MemoryBlock {
size_t start;
size_t size;
bool free;
MemoryBlock(size_t start, size_t size, bool free = true)
: start(start), size(size), free(free) {}
};
class MemoryAllocator {
public:
MemoryAllocator(size_t totalSize) {
blocks.push_back(MemoryBlock(0, totalSize));
}
void* allocate(size_t size) {
for (auto& block : blocks) {
if (block.free && block.size >= size) {
block.free = false;
if (block.size > size) {
blocks.push_back(MemoryBlock(block.start + size, block.size - size));
block.size = size;
}
std::cout << "Allocated " << size << " bytes at address " << block.start << std::endl;
return reinterpret_cast<void>(block.start);
}
}
std::cout << "Failed to allocate " << size << " bytes" << std::endl;
return nullptr;
}
void deallocate(void* ptr) {
auto it = std::find_if(blocks.begin(), blocks.end(),
[ptr](const MemoryBlock& block) { return reinterpret_cast<void>(block.start) == ptr; });
if (it != blocks.end()) {
it->free = true;
std::cout << "Deallocated " << it->size << " bytes at address " << it->start << std::endl;
mergeAdjacentFreeBlocks();
} else {
std::cout << "Invalid pointer for deallocation" << std::endl;
}
}
private:
std::vector<memoryblock> blocks;
void mergeAdjacentFreeBlocks() {
std::sort(blocks.begin(), blocks.end(),
[](const MemoryBlock& a, const MemoryBlock& b) { return a.start < b.start; });
for (auto it = blocks.begin(); it != blocks.end(); ) {
auto next = std::next(it);
if (next != blocks.end() && it->free && next->free) {
it->size += next->size;
blocks.erase(next);
} else {
++it;
}
}
}
};
int main() {
MemoryAllocator allocator(1024); // 1024 bytes total memory
void* ptr1 = allocator.allocate(256);
void* ptr2 = allocator.allocate(128);
void* ptr3 = allocator.allocate(512);
allocator.deallocate(ptr2);
allocator.deallocate(ptr1);
void* ptr4 = allocator.allocate(384);
allocator.deallocate(ptr3);
allocator.deallocate(ptr4);
return 0;
}
</memoryblock></void></void>
이 메모리 할당기는 First-Fit 알고리즘을 사용합니다. 즉, 요청된 크기를 수용할 수 있는 첫 번째 자유 블록을 찾아 할당합니다. 또한, 메모리 단편화를 줄이기 위해 인접한 자유 블록들을 병합하는 기능도 구현되어 있습니다.
이러한 메모리 관리 기법은 실제 운영체제에서 사용되는 방식과 유사합니다. 물론 실제 운영체제는 더 복잡하고 효율적인 알고리즘을 사용하지만, 이 예제를 통해 기본적인 개념을 이해할 수 있습니다.
3. 파일 시스템: 간단한 가상 파일 시스템 구현 📁
파일 시스템은 운영체제의 또 다른 중요한 구성 요소입니다. 여기서는 간단한 가상 파일 시스템을 C++로 구현해보겠습니다. 이 시스템은 기본적인 파일 생성, 읽기, 쓰기, 삭제 기능을 지원합니다.
#include <iostream>
#include <unordered_map>
#include <string>
#include <vector>
#include <stdexcept>
class File {
public:
File(const std::string& name) : name(name) {}
void write(const std::string& content) {
this->content = content;
}
std::string read() const {
return content;
}
std::string getName() const {
return name;
}
private:
std::string name;
std::string content;
};
class Directory {
public:
Directory(const std::string& name) : name(name) {}
void addFile(const std::string& fileName) {
files[fileName] = File(fileName);
}
File& getFile(const std::string& fileName) {
if (files.find(fileName) == files.end()) {
throw std::runtime_error("File not found");
}
return files[fileName];
}
void deleteFile(const std::string& fileName) {
if (files.find(fileName) == files.end()) {
throw std::runtime_error("File not found");
}
files.erase(fileName);
}
std::vector<:string> listFiles() const {
std::vector<:string> fileList;
for (const auto& pair : files) {
fileList.push_back(pair.first);
}
return fileList;
}
std::string getName() const {
return name;
}
private:
std::string name;
std::unordered_map<:string file> files;
};
class FileSystem {
public:
void createDirectory(const std::string& dirName) {
directories[dirName] = Directory(dirName);
}
Directory& getDirectory(const std::string& dirName) {
if (directories.find(dirName) == directories.end()) {
throw std::runtime_error("Directory not found");
}
return directories[dirName];
}
void deleteDirectory(const std::string& dirName) {
if (directories.find(dirName) == directories.end()) {
throw std::runtime_error("Directory not found");
}
directories.erase(dirName);
}
std::vector<:string> listDirectories() const {
std::vector<:string> dirList;
for (const auto& pair : directories) {
dirList.push_back(pair.first);
}
return dirList;
}
private:
std::unordered_map<:string directory> directories;
};
int main() {
FileSystem fs;
fs.createDirectory("documents");
fs.createDirectory("pictures");
Directory& docs = fs.getDirectory("documents");
docs.addFile("report.txt");
docs.getFile("report.txt").write("This is a report");
Directory& pics = fs.getDirectory("pictures");
pics.addFile("vacation.jpg");
std::cout << "Directories: ";
for (const auto& dir : fs.listDirectories()) {
std::cout << dir << " ";
}
std::cout << std::endl;
std::cout << "Files in documents: ";
for (const auto& file : docs.listFiles()) {
std::cout << file << " ";
}
std::cout << std::endl;
std::cout << "Content of report.txt: " << docs.getFile("report.txt").read() << std::endl;
docs.deleteFile("report.txt");
fs.deleteDirectory("pictures");
std::cout << "Directories after deletion: ";
for (const auto& dir : fs.listDirectories()) {
std::cout << dir << " ";
}
std::cout << std::endl;
return 0;
}
</:string></:string></:string></:string></:string></:string>
이 가상 파일 시스템은 디렉토리와 파일의 기본적인 구조를 모방합니다. Directory 클래스는 파일들을 관리하고, FileSystem 클래스는 디렉토리들을 관리합니다. 각 파일은 이름과 내용을 가지며, 읽기와 쓰기 작업을 수행할 수 있습니다.
이러한 구조는 실제 파일 시스템의 기본적인 개념을 반영하고 있습니다. 물론 실제 파일 시스템은 더 복잡하고 효율적인 구조를 가지고 있지만, 이 예제를 통해 파일 시스템의 기본 개념을 이해할 수 있습니다.
4. 프로세스 간 통신(IPC): 파이프 구현 🔗
프로세스 간 통신(Inter-Process Communication, IPC)은 운영체제에서 중요한 개념 중 하나입니다. 여기서는 가장 기본적인 IPC 메커니즘 중 하나인 파이프를 C++로 구현해보겠습니다.
#include <iostream>
#include <vector>
#include <thread>
#include <mutex>
#include <condition_variable>
#include <queue>
template<typename T>
class Pipe {
public:
void write(const T& data) {
std::unique_lock<std::mutex> lock(mutex);
buffer.push(data);
cv.notify_one();
}
T read() {
std::unique_lock<std::mutex> lock(mutex);
cv.wait(lock, [this] { return !buffer.empty(); });
T data = buffer.front();
buffer.pop();
return data;
}
private:
std::queue<T> buffer;
std::mutex mutex;
std::condition_variable cv;
};
void producer(Pipe<int>& pipe) {
for (int i = 0; i < 10; ++i) {
pipe.write(i);
std::cout << "Produced: " << i << std::endl;
std::this_thread::sleep_for(std::chrono::milliseconds(100));
}
}
void consumer(Pipe<int>& pipe) {
for (int i = 0; i < 10; ++i) {
int data = pipe.read();
std::cout << "Consumed: " << data << std::endl;
std::this_thread::sleep_for(std::chrono::milliseconds(150));
}
}
int main() {
Pipe<int> pipe;
std::thread producerThread(producer, std::ref(pipe));
std::thread consumerThread(consumer, std::ref(pipe));
producerThread.join();
consumerThread.join();
return 0;
}
이 예제에서는 템플릿을 사용하여 다양한 데이터 타입을 지원하는 파이프를 구현했습니다. 파이프는 내부적으로 큐를 사용하여 데이터를 저장하고, 뮤텍스와 조건 변수를 사용하여 동기화를 처리합니다.
producer 함수는 데이터를 생성하여 파이프에 쓰고, consumer 함수는 파이프에서 데이터를 읽어 처리합니다. 이 두 함수는 별도의 스레드에서 실행되어 병렬 처리를 시뮬레이션합니다.
이러한 IPC 메커니즘은 실제 운영체제에서 프로세스 간 데이터 교환과 동기화에 사용됩니다. 예를 들어, 유닉스 시스템의 파이프 명령어(|)가 이와 유사한 개념으로 동작합니다.
5. 스레드 관리: 스레드 풀 구현 🧵
현대의 운영체제는 멀티스레딩을 효율적으로 관리하기 위해 스레드 풀을 사용합니다. 여기서는 간단한 스레드 풀을 C++로 구현해보겠습니다.